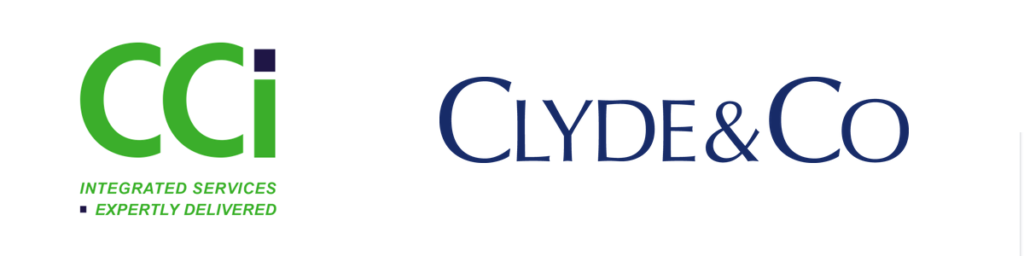
Emerging from the grip of a global pandemic, the world faces unprecedented times. Times that have challenged the global society about their attitudes and perceptions of key issues. But those key issues are in a constant state of flux and are ever-changing. One area that has been pushed to the fore is Environmental Social Governance (ESG). The ESG agenda has made both individuals and companies alike question how energy is consumed, but perhaps more significantly, how it is generated.
Accordingly, the world finds itself in the midst of an energy transition driven by our choices of what is deemed to be an acceptable impact on the environment in view of global energy needs. This transition has led to a dramatic growth in the installation of renewable power sources, corresponding with the increasingly ambitious growth of governmental environmental targets. A vital component of the growing number of renewable power sources seeking to meet these ambitious targets is wind.
The wind industry can be broadly split into three areas, namely: onshore, fixed bottom offshore and floating offshore. Undoubtedly onshore is the most mature of these markets accounting for 95% of the 743GW[1] installed globally to date. Offshore wind, whilst some way behind, is seeing historic growth, not least because approximately 80% of the world’s potential wind resource is offshore in deep water.[2]
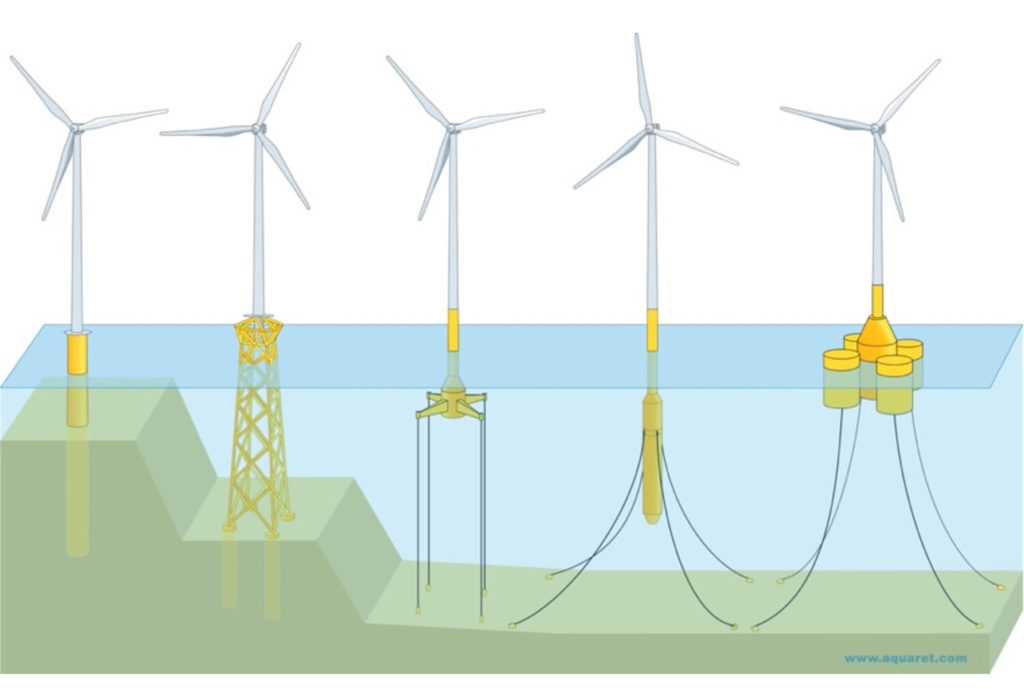
Historically, it was broadly accepted that 50m was the deep water tipping point from the limit of fixed bottom to the viability of floating. However, the UK Department for Business, Energy, & Industrial Strategy (BEIS) recently announced changes to the Contract for Difference (CfD) scheme to allow for a separate strike price for Floating Offshore Wind (FOW) projects at water depths greater than 60m,[5] indicating that the tipping point should be adjusted.
Either depth restriction greatly limits the range of locations suitable for fixed bottom offshore wind development. In short, indications are that to exploit the full wind potential of offshore wind, we must look to floating wind.
Common challenges to all floating wind
As water depth increases, it becomes more difficult, disproportionately costly and risky to fabricate and install fixed foundations. However, floating foundations have the potential to provide a solution. In water depths beyond 60m floating wind opens up higher resource sights, offers a potentially lower cost alternative resulting from standardised designs, and installation can be done using readily available, lower cost vessels.
Unfortunately, this ideal is some way off. It is forecast that FOW Levelised Cost of Energy (LCoE)[8] levels will not reach parity with fixed bottom wind until early in the next decade.[9] Key to the reduction of LCoE for FOW will be the adoption at scale of the technology, which in turn will drive standardisation of designs and result in cost efficiencies. The challenges for the supply chain will be to achieve the necessary cost reductions by:
- Making efficiencies in the design(s) to reduce structural mass;
- Developing modular designs that can be fabricated in series;
- Developing designs which account for the general risks associated with offshore wind installations as well as the risks specific and particular to floating foundations;
- Developing integrated, coupled analysis models for foundations and turbines to optimise overall system performance; and
- Optimising the installation processes for multiple floating installations for commercial scale farms.
Whilst the consensus is that FOW could be a potential lower cost alternative in deeper waters, currently that is not the case. As things stand, floating foundations are estimated to be 1.5 – 2 times more expensive than fixed bottom foundations on a like-for-like basis.
There are currently more than 40 floating foundation concepts in existence, and the industry needs to decide which of these are viable for large-scale commercial development. The relevance of a particular design will vary from site-to-site depending on the water depth, seabed morphology, prevailing sea conditions, local infrastructure and local supply chain capabilities. However, for ease of comparison, floating foundations can be grouped into three general categories, namely: spar, semi-submersible, and Tension Leg Platform (TLP).
These are all technologies that have been carried over from the upstream energy sector and have been used for many years with great success. The design of floating foundations can therefore draw upon the wealth of experience gained during the development of upstream oil and gas assets, as well as fixed bottom offshore wind foundations. However, when making sector comparisons, we should be aware of some key differences. An oil and gas facility is often a bespoke, one-off installation and is not subject to the efficiency considerations needed for repeat fabrication, transportation and installation operations seen in a wind farm. Second, there are significant differences in size. For example, the Aasta Hansteen Floating Production Storage and Offloading (FPSO) platform is a spar hull at 50m in diameter and 198m in length. By comparison, the Hywind Tampen spar hulls are 18.8m in diameter and 107.5m in length. This differential gives rise to many issues and considerations peculiar to FOW, for example, the comparative lack of space available between the mooring and cable systems in the FOW foundations required to avoid clashing during excursions of the turbine in response to the seaway.
Another consideration relates to operation and maintenance. The risks associated with mooring chain/ mooring line failure are well-known in the oil and gas insurance market. Mooring chains can fail if there are defects in the metal or in the welding, and chains/ lines can corrode (especially in the splash zone) if they are not properly maintained. Whilst oil and gas operators have sought to mitigate this risk by regular inspection of lines and chains, failures do still happen. This risk could be greater for FOW foundations given that (i) grid-scale FOW projects will have tens of individual floating foundations, multiplying the risk considerably compared to a single FPSO or moored drillship for example, and (ii) unlike an FPSO or moored drillship, FOW projects will not be permanently crewed which potentially makes routine maintenance more challenging. Whilst the possibility is remote, the risk of a floating wind foundation breaking completely free of its moorings and damaging surrounding assets cannot be entirely ruled out.
Ultimately, considerable research and development is required to ensure that the designs of floating foundations are capable of mitigating and/or avoiding the risks involved with this new and innovative technology.
Comparing hull types
While FOW is the next evolution of the wind industry, many factors will dictate which of the three categories will become dominant. A recurring theme in this comparison is the motion response of each type. Given that turbine nacelles are particularly susceptible to accelerations, considering how the hull will respond to the seaway and how that motion can be controlled is crucial. What follows is a description of each type, followed by a summary of the principal strengths and weaknesses.
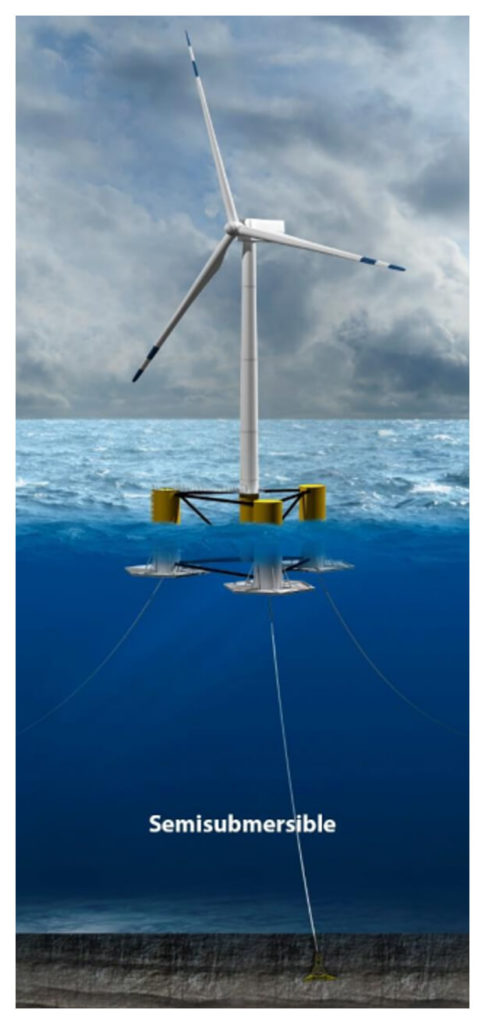
Semi-Submersible
A semi-submersible foundation achieves stability from a wide buoyancy spread at the water plane. Their motion response is moderate in pitch and roll but significantly more in heave than other concepts, due to the increased buoyancy of the hull over a relatively large surface area.
- Easy to install, with broad weather windows
- Can be towed to location
- Comparatively simple mooring system lowers overall risk and cost
- Specialist installation & decommissioning vessels not required
- road water depth suitability
- Soil condition insensitivity
- Tendency for higher critical wave-induced motions requiring some form of damping
- Larger, more complex structures tend to use more material compared to other concepts
- Complexity of structure makes fabrication labour intensive, with long lead times
- Considerable lateral excursions present potential clash issues for cables
- Fabricated as a single structure, requiring dry dock or load out skids at yard
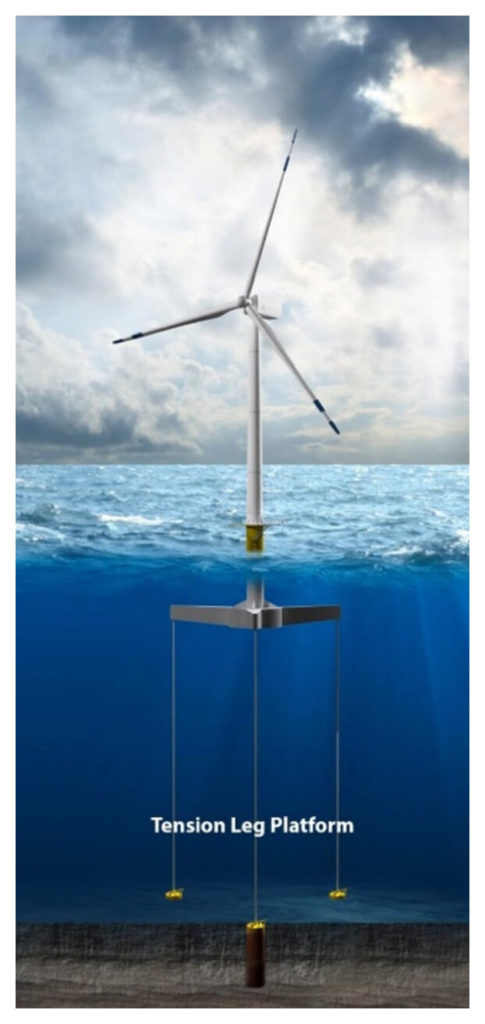
TLP
A TLP achieves its stability as a result of high tension in the individual tendon legs, created by the highly buoyant hull. These taunt legs limit motions, giving attractive dynamics, yet they are not broadly adopted.
- Tendency for lower critical wave-induced motions in pitch, roll and heave
- High stability from tension legs
- Low hull mass, also meaning lower material costs
- Suitable for the broadest range of water depths
- Small seabed footprint
- Simple, light structure
- Low stability during transportation and installation
- High vertical loads on moorings
- Complex, costly installation operation to connect legs
- Reliance on taunt legs for stability presents higher operational risk levels in the event of single leg failure
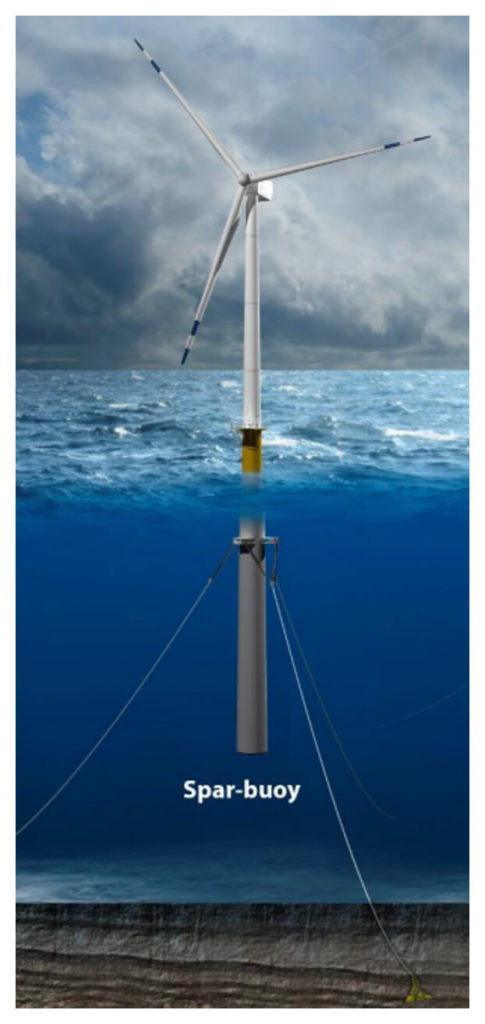
Spar buoy
Spar buoys are cylindrical hulls with a low water plane area that are stabilised by heavy ballast in the lower hull below the centre of gravity. Additionally, the large weight low in the hull limits heave response, but the elongated shape of the spar design can make it more susceptible to pitch and roll motions.
- Tend to have lower critical wave-induced motions
- Relatively simple hull to construct
- Suitable for higher sea states
- Can be towed to location
- Comparatively simple mooring system lowers overall risk and cost
- Low operational risk as inherently stable
- Soil condition insensitivity
- Heavy, long hull requires deep-water fabrication and installation site
- Deep draught limits fabrication yards
- Higher than average fatigue loads in tower base
- Mating of turbine and hull have to be conducted in relatively sheltered waters
- Large mooring seabed foot print
Conclusion
With approximately 80% of the world’s resource of strong wind being located in deep water, FOW is the technology with the potential to unlock it. However, it is likely to be at least a decade before FOW is at parity with fixed bottom wind in terms of cost. The barriers to achieving parity with fixed bottom wind include:
- Supply chain development for industrialisation at scale;
- Selection of leading concepts;
- Acceleration towards commercial scale projects;
- Resolving the fabrication to installation challenge;
- Achieving cost reduction through standardisation, modularisation, increase of technology readiness level, insurance and lowering the cost of project finance; and
- Favourable floating specific regulatory frameworks.
As a result, no one particular technology type presents a clear advantage at present. Therefore until such time as the industry has evolved and experience gained, each project must be considered on the relative merits of its hull concept selection, and the chosen installation, operation and maintenance methodologies.
There are undoubtedly risks associated with FOW, not least the challenge of monitoring the condition of a very large number of mooring lines/ mooring chains at each project, but there are also possible upsides from an insurance perspective. For example, it may be possible to tow turbines to a port to conduct repairs rather than having to use expensive heavy lift vessels to conduct repairs offshore, meaning repairs can be conducted at lower cost and (possibly) in higher sea states thereby getting power back on line quicker and reducing business interruption outages.
There is no question that we will see huge growth in grid-scale floating offshore wind projects in the coming decade, and whatever foundation model is eventually favoured, developers and operators will need to pay close attention to how risk can best be minimised.
Authors
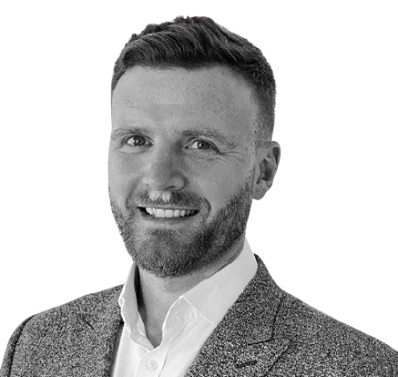
Renewables Lead
London
+44 (0)7730 445 020
Daniel.Sim@ccint.co.uk
CCi
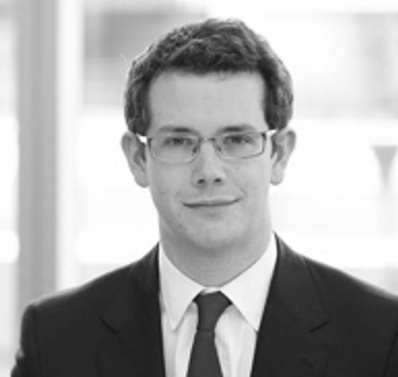
London
+44 20 7276 4435
Paul.Lowrie@clydeco.com
Clyde & Co LLP
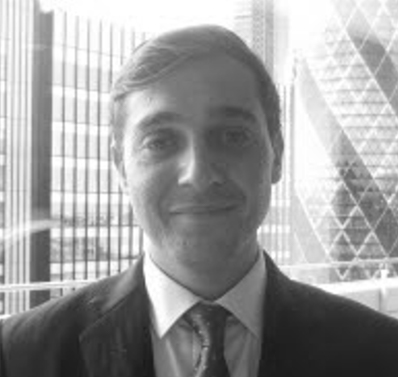
London
+44 20 7876 6086
Max.Braslavsky@clydeco.com
Clyde & Co LLP